A capacitive load must affect the performance of an operational amplifier. Simply put, a capacitive load can turn an amplifier into an oscillator. Today we will talk about –
How a capacitive load can turn an amplifier into an oscillator
How to handle capacitive loads?
Amplifiers into oscillators? There’s a principle to it!
The output resistance Ro inherent to an operational amplifier, together with the capacitive load, forms another pole of the amplifier transfer function. As shown in the Porter diagram, at each pole the amplitude slope (negative value) decreases by 20 dB/10 times. Note how each pole increases the phase shift by as much as -90°. We can examine the instability problem from two perspectives. Look at the amplitude response on the log plot, where the circuit becomes unstable when the sum of the open loop gain and feedback attenuation is greater than 1. Similarly, look at the phase response, where the op-amp tends to oscillate at frequencies where the loop phase shift exceeds -180°, if this frequency is below the closed-loop bandwidth. The closed-loop bandwidth of a voltage feedback op-amp circuit is equal to the gain bandwidth product of the op-amp (GBP, or unit gain frequency) divided by the closed-loop gain of the circuit (ACL).

The phase margin of an operational amplifier circuit can be thought of as the additional phase shift in the closed-loop bandwidth required to make the circuit unstable (i.e., phase shift + phase margin = -180°). As the phase margin tends to zero, the loop phase shift tends to -180° and the op-amp circuit becomes unstable. In general, if the phase margin value is much less than 45°, it can lead to spikes in the frequency response and problems such as overshoot or ringing oscillations in the step response. To maintain sufficient phase margin, the poles generated by the capacitive load should be at least 10 times higher than the closed-loop bandwidth of the circuit. If this is not the case, consider the possibility of circuit instability.
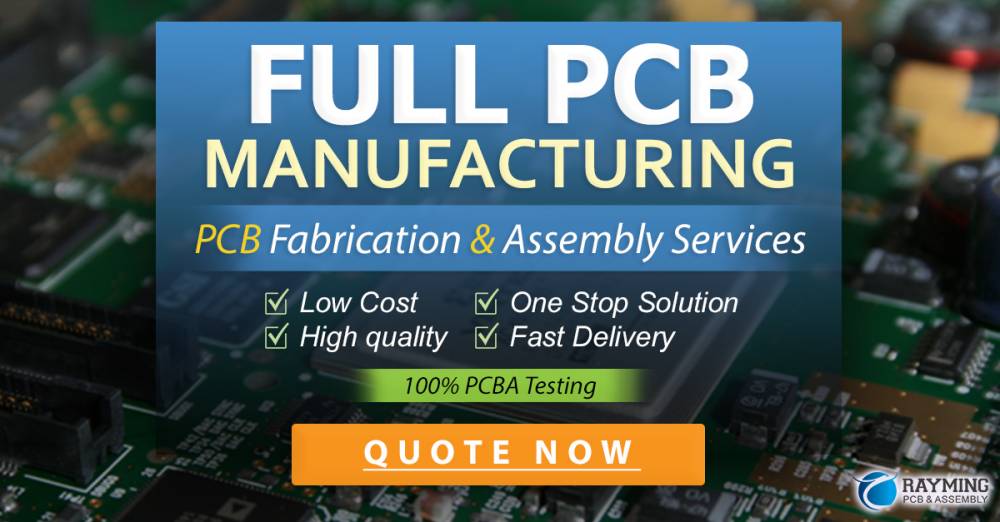
How to handle capacitive loads? Teach you three tricks
The first step should be to determine if the op-amp can safely drive its own load. Many op amp datasheets specify “capacitive load drive capability”, while others provide typical data on “small signal overshoot versus capacitive load”. Looking at these values, one can see that overshoot increases exponentially with increasing load capacitance. As the overshoot approaches 100%, the op amp becomes unstable. If possible, keep the overshoot well below this limit. Also note that this graph is for a specific gain. For voltage feedback op amps, the capacitive load drive capability increases as the gain increases. Therefore, a voltage feedback op amp that can safely drive a 100pF capacitor at unit gain should be able to drive a 1000pF capacitor at a gain of 10.
Some op-amp data sheets give the open-loop output resistance (Ro), from which the frequency of the additional pole mentioned above can be calculated. If the frequency of the additional pole (fp) is 10 times higher than the circuit bandwidth, the circuit will remain stable.
If the data sheet for the op-amp does not state the capacitive load driving capability or the open loop output resistance, and does not provide a graph of overshoot versus capacitive load, then it must be assumed that any load capacitance requires some sort of compensation technique in order to ensure stability. There are many ways to make a standard op-amp circuit stable to drive a capacitive load, several of which are listed below.
01 Noise Gain Manipulation
This is an effective method for maintaining stability in low frequency applications, yet is often overlooked by designers. The principle is to increase the closed-loop gain of the circuit (also called “noise gain”) without changing the signal gain, thereby reducing the frequency at which the product of open-loop gain and feedback attenuation becomes one. This can be achieved by connecting RD between the inputs of operational amplifiers in some circuits, as shown in the figure below. Using the given formula, the “noise gain” of these circuits can be found.

Since stability is controlled by noise gain rather than signal gain, the above circuit improves stability without affecting signal gain. Stability is ensured by simply making the “noise bandwidth” (GBP/ANOISE) at least 10 times lower than the pole generated by the load.

This stabilization method has the disadvantage that the voltage noise and input detuning voltage folded into the input are further amplified, resulting in increased output noise and detuning voltage. Connecting the capacitor CD in series with the RD eliminates the increased DC bias voltage, but this technique increases the noise and cannot be eliminated. The effective noise gain of these circuits with and without CD is shown in the figure.
When used, CD should be as large as possible; the minimum value should be 10ANOISE/(2πRDGBP) in order to make the “noise pole” at least 10 times lower than the “noise bandwidth”.
02 Out-of-loop compensation
This method is to add a resistor RX between the output of the op-amp and the load capacitor, as shown in the figure below. This resistor is obviously outside the feedback loop, but together with the load capacitor, it leads a zero point into this transfer function of the feedback network, thus reducing the phase shift of the loop at high frequencies.

To ensure stability, the RX value should be such that the increased zero point (fZ) is at least 10 times lower than the closed-loop bandwidth of the op-amp circuit. By increasing RX, the circuit performance will not be affected as in the first method, and the output noise will not increase, but the output impedance relative to the load will increase. Since RX and RL form a resistive voltage divider, this may reduce the signal gain. If RL is known and fairly stable, the gain of the op-amp circuit can be increased to offset this gain loss.
This method is very effective for driving transmission lines. To avoid standing waves, the values of RL and RX must be equal to the characteristic impedance of the cable (typically 50Ω or 75Ω). Therefore, RX is predetermined and the rest of the work is to double the gain of the amplifier in order to offset the signal loss caused by the resistive divider, and the problem is solved.
03 In-loop compensation
If RL is unknown or dynamically varying, the effective output resistance of the gain stage must be kept low. In this case, it may be helpful to connect the RX within the entire feedback loop, as shown in the figure below. With this configuration, the DC and low frequency counter do come from the load itself, so the signal gain from the input to the load remains unaffected by the voltage divider (RX and RL).

The added capacitor CF in this circuit cancels out the poles and zeros caused by CL. Simply put, the zero point created by CF coincides with the pole created by CL, while the pole created by CF coincides with the zero point created by CL. As a result, the total transfer function and phase response are exactly the same as they would be without the capacitor. To ensure that both the pole and zero combinations cancel, the above equations must be solved exactly. Additional care should be taken with the conditions; if the load impedance is relatively large, these conditions are easily satisfied.
If the RO is unknown, it will be difficult to calculate. In this case, the design procedure becomes a guessing game, which can be a nightmare for circuit design. One thing should be noted about SPICE: the SPICE model of the op-amp does not accurately model the open-loop output resistance (RO), so it does not completely replace the empirical design of the compensation network.
It is also important to note that the CL must be a known and constant value for this technique to be applied. Many applications have amplifiers driving unconventional loads and the CL may vary significantly depending on the load. Using the above circuit is only optimal when CL is part of a closed loop system.
One application is to buffer or invert the reference voltage to drive a larger decoupling capacitor. In this case, the CL is a fixed value and can precisely offset the pole/zero combination. The low DC output impedance and low noise (compared to the previous two methods) of this method are very favorable. In addition, the decoupling capacitance of the reference voltage can be large (often a number of microfarads), using other compensation methods is not feasible.
All three of these methods are used in “standard”, unit gain stabilized, voltage feedback op amps, each with its own advantages and disadvantages. Now, you can apply your knowledge to determine which method is best for your application!