This is called the DC bias of operational amplifier, if the amplification is large, this DC bias will also be amplified, in order to eliminate the DC bias, add a resistor of several M at the power supply and input of the op-amp, or some op-amps themselves have a zeroing terminal Voffset, connected to a resistor This resistor is called a bias resistor.
The core component of the amplifier circuit is the transistor, so it is necessary to have a certain understanding of the transistor. There are many types of amplification circuits with transistors, so let’s explain them with some common ones. Figure 1 shows the basic amplification circuit of a total shot. What do we generally need to know about amplification circuits?
(1) Analyze the role of each component in a circuit;
(2) To liberate the amplification principle of a large circuit;
(3) Analyze and calculate the static operating point of a circuit;
(4) understand the purpose and method of setting the static operating point.
Of the above four items, the last one is more important.

Calculation of bias resistance
Figure 1, C1, C2 is the coupling capacitor, coupling is to play a signal transfer role, the capacitor can be coupled from the front signal signal to the rear, because the voltage at both ends of the capacitor can not change abruptly, after the input AC signal at the input, because the voltage at both ends can not change abruptly due to, the voltage at the output will follow the input AC signal at the input, thus coupling the signal from the input to the output. But one point to clarify is that the voltage at both ends of the capacitor can not change abruptly, but not can not change.
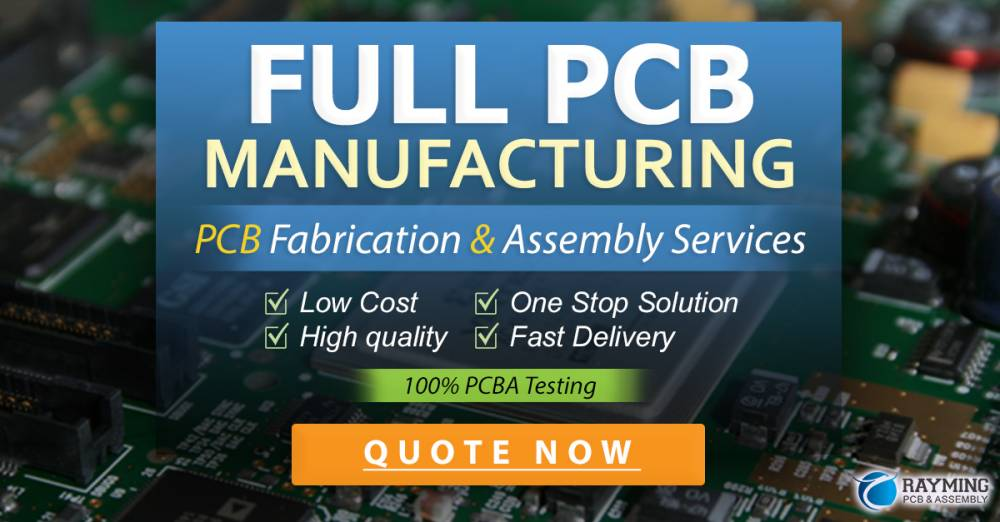
R1, R2 for the triode V1 DC bias resistance, what is meant by DC bias? Simply put, do the work to eat. Requires the transistor to work, must first provide certain working conditions, electronic components must be required to have electrical power supply, otherwise it is not called a circuit.
In the working requirements of the circuit, the first condition is required to be stable, so the power supply must be DC power, so called DC bias. Why is the power supplied through a resistor? The resistor is like a faucet in a water supply system, used to regulate the amount of current. Therefore, the three working states of the transistor “: carrier stop, saturation, amplification” is determined by the DC bias, in Figure 1, that is, by R1, R2 to decide.
First of all, we need to know how to identify the three working states of the transistor, in short, to identify the work in what working state can be based on the size of Uce to identify, Uce close to the supply voltage VCC, the transistor will work in the carrier state, carrier state is to say that the transistor basically does not work, Ic current is small (about zero), so R2 due to no current flow, the voltage is close to 0V, so Uce is close to the supply voltage VCC. So Uce is close to the supply voltage VCC.
If Uce is close to 0V, the triode works in saturation, what is saturation? That is, the Ic current has reached its maximum value, even if Ib increases, it can no longer increase.
The above two states we generally referred to as the switching state, in addition to these two, the third state is the amplification state, the general measurement of Uce is close to half of the supply voltage. If the measurement of Uce bias to VCC, the transistor tends to the carrier state, if the measurement of Uce bias to 0V, the transistor tends to saturated state.
Understand the purpose and method of setting the static operating point
Amplification circuit, is the input signal amplification and output, (generally there are voltage amplification, current amplification and power amplification several, this is not in this discussion). Let’s start with the signal we want to amplify, and take the sinusoidal AC signal as an example. In the analysis process, you can only take into account the signal size change is positive and negative, other than to say. Above mentioned in Figure 1 amplifier circuit circuit, the static operating point is set to Uce close to half of the supply voltage, why?
This is to make the signal positive and negative can have a symmetrical change space, when there is no signal input, that is, the signal input is 0, assuming that Uce is half of the supply voltage, we when it is a horizontal line, as a reference point. When the input signal increases, then Ib increases, Ic current increases, then the voltage of the resistor R2 U2 = Ic & TImes; R2 will increase, Uce = VCC – U2, will become smaller. U2 maximum theoretical can reach equal to VCC, then Uce minimum will reach 0V, this is to say, in the input letter increases, Uce maximum change from 1/2 of VCC change to 0V.
Similarly, when the input signal decreases, then Ib decreases, Ic current decreases, then the voltage of the resistor R2 U2 = Ic & TImes; R2 will then decrease, Uce = VCC – U2, will become larger. In the input letter decreases, Uce maximum change from 1/2 VCC change to VCC. so that the input signal within a certain range of positive and negative changes, Uce to 1/2 VCC prevail then there is a symmetrical range of positive and negative changes, so the general Figure 1 static operating point is set to Uce close to half of the supply voltage.
To design Uce to be close to half of the supply voltage, which is our purpose, but how can Uce be designed to be close to half of the supply voltage? This is the means to do so.
Here to know a few things, the first is that we often say Ic, Ib, they are the collector current and base current of the transistor, they have a relationship is Ic = β & TImes; Ib, but when we first learn, the teacher obviously did not tell us, Ic, Ib is how big to be appropriate? This question is difficult to answer, because there are more things involved, but in general, for small power tubes, generally set Ic in the zero point a few milliamps to a few milliamps, medium power tubes are in a few milliamps to tens of milliamps, high-power tubes are in the tens of milliamps to a few amps.
In Figure 1, set Ic for 2mA, then the resistance value of the resistor R2 can be calculated by R = U / I, VCC for 12V, then 1/2 VCC for 6V, R2 resistance value of 6V/2mA, for 3KΩ. Ic set to 2mA, then Ib can be launched by Ib = Ic / β, the key is the value of β, β general theory to take the value of 100, then Ib = 2mA / 100 = 20 # A, then R1 = (VCC-0.7V)/Ib = 11.3V/20#A = 56.5KΩ, but in fact, the β value of small power tubes is much more than 100, between 150 and 400, or higher, so if the above calculation to do, the circuit is likely to be in saturation, so sometimes we do not understand that the calculation is correct, but the actual can not be used, it is because there is also a little less Practical guidance, pointing out the difference between theory and practice. This circuit is greatly influenced by the value of β. When each person calculates the same, but the result is not necessarily the same. In other words, the stability of this circuit is poor, and there are fewer practical applications. However, if it is changed to the voltage divider bias circuit in Figure 2, the analytical calculation of the circuit and the actual circuit measurement are closer.

Calculation of bias resistance
In the voltage divider bias circuit in Figure 2, again we assume that Ic is 2mA and Uce is designed to be 1/2VCC for 6V. Then how should R1, R2, R3, and R4 be valued. The formula is as follows: Because Uce is designed to 1/2VCC is 6V, then Ic & TImes; (R3 + R4) = 6V; Ic ≈ Ie. can be calculated R3 + R4 = 3KΩ, so that R3, R4 is how much each?
General R4 take 100Ω, R3 for 2.9KΩ, in fact R3 we generally straight take 2.7KΩ, because the E24 series resistors do not have 2.9KΩ, take the value of 2.7KΩ and 2.9KΩ is no big difference. Because the voltage across R2 is equal to Ube + UR4, that is, 0.7V + 100Ω × 2mA = 0.9V, we set Ic for 2mA, β general theory to take the value of 100, then Ib = 2mA / 100 = 20 # A, there is a current to estimate, is the current flowing through R1, generally take the value of Ib about 10 times, take IR1200 # A. Then R1 = 11.1V/200#A≈56KΩR2=0.9V(/200-20)#A=5KΩ;Considering that the actual value of β may be much larger than 100, so the actual value of R2 is 4.7KΩ.Thus, the values of R1, R2, R3, R4 are 56KΩ, 4.7KΩ, 2.7KΩ, 100Ω, and Uce is 6.4V.
In the above analysis and calculation, many times the assumptions and what not, which is necessary in the practical application, many times need a reference value to give us calculations, but often do not, which is one we are not familiar with the various devices, the second is to forget one thing, we ourselves are the ones who use the circuit, some data can be set by themselves, so you can take the road less traveled.
Calculation of cathode bias resistance and feedback resistance?
For amplifiers with large loop negative feedback applied at the cathode of the input stage, the EP2C8F256CXNAA calculation of the cathode bias resistance and feedback resistance is easily the most complex calculation in the design process. However, if we can keep our composure and get all the information well labeled by drawing numerous concise circuit analysis diagrams, the problem can be simplified and can reach a level we can control.
It is difficult to get an answer by just writing on the back of an envelope. We need to consider the following 4 main factors at the same time.
-We need to set the cathode bias voltage correctly. Normally, this is a simple application of Ohm’s Law; but here it is slightly more complicated because the bias current will flow through both the cathode resistor and the feedback resistor.
-The tube itself generates current feedback in the cathode resistor, and this cathode resistor, in turn, has current flowing through it from the output of the amplifier.
-We need to set the ratio of the cathode resistor to the feedback resistor in order to get the desired amount of negative feedback.
-In the case of AC, which is our concern, the cathode resistor is connected in parallel with the ‰ of the input tube. We already know the limiting factors and now, it should be possible to draw a diagram for labeling and do some calculations using the formula.
Since we need to make the cathode voltage equal to 2.5V and the anode current 190V/47kQ, the total resistance between the cathode and ground must be 618.4Q.
This means that the anode signal current must be 8.636V/47kQ=0.1837mARMs. This current also flows into the cathode circuit and forms a feedback voltage on the resistor that is not bypassed.
We want the input sensitivity of this amplifier to be 2Ms. We also know that the input sensitivity is 298Ms before applying a large loop negative feedback. therefore, the feedback voltage at the cathode needs to be 2V a 0.298V = 1.702Ms. we know that the output signal of the amplifier will be 8.944Ms at an output of 10W.